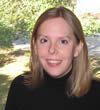
Dwyer, Noelle
Primary Appointment
Associate Professor, Cell Biology
Education
- BA, Biochemistry, Rice University
- PhD, Neuroscience, University of California, San Francisco
- Postdoc, Neurodevelopment, The Salk Institute
- Postdoc, Neurogenetics, Beth Israel Hospital, Boston, MA
Contact Information
PO Box 800732
Department of Cell Biology
Charlottesville, VA
Telephone: 2-0692
Email: ndd9b@virginia.edu
Research Disciplines
Cancer Biology, Cell and Developmental Biology, Development, Stem Cells & Regeneration, Genetics, Molecular Biology, Neuroscience, Stem Cells & Regeneration
Research Interests
Neural Development; Cell Division in Neural Stem Cells; Axon Outgrowth and Guidance
Research Description
Development of the Cerebral Cortex
Your cerebral cortex mediates your conscious sensory perceptions, thoughts, and behaviors. To perform these functions, the cortex must develop the proper structure and circuitry during early life. In the embryo, the cortex begins as an epithelial sheet of neural stem cells. The sheet expands and balloons outward due to symmetric divisions of these polarized cells. Later, the stem cells divide asymmetrically, eventually generating billions of neurons and glia. The neurons and glia form a six-layered structure with different areas specialized for functions such as vision or hearing. Finally, neurons within each area must be "wired" to other specific brain regions through axonal connections. The genetic and cellular mechanisms that control all these events are only beginning to be understood, and comprise some of the most compelling mysteries of biology and medicine. How is the correct size of the cerebral cortex specified? What are the changes in neural development that underlie brain malformations, seizures, intellectual disability, schizophrenia, or autism?
The long-term goal of our lab is to help answer these questions, and elucidate fundamental cellular mechanisms important not only in the nervous system, but in development of many tissues and disease processes. In particular, we have been using genetics in mice to elucidate mechanisms of early cortical development. We study mutants with specific defects in brain growth and wiring. By studying the brain phenotypes and the cellular roles of the encoded proteins, with both in vivo and in vitro experiments, we hope to understand how small changes at the single-cell level can cause dramatic changes in overall brain structure. Our novel gene discoveries have led to cellular and molecular studies on topics such as cell division, cytokinesis, neuron polarization, and axon guidance. We are continuing to identify new mouse mutants that affect brain growth and architecture.
Neurogenesis: how cell division mechanisms produce different daughter cells at different times to build a proper brain
The highly polarized structure of neuroepithelial stem cells places special constraints on the cell cycle and mitosis. The stem cells must make a faithful copy of their genome, and then split in half while maintaining their polarity and attachments within the epithelial sheet. They must also produce daughter cells in the right order: first more stem cells, then neurons, and finally glia. We are studying the mechanisms of these unusual cell divisions, and how the way that the cells divide influences the structure and fate of daughter cells. When the cells split in two during cytokinesis, the cleavage furrow completes with a final bridge between the two cells called the midbody. This bridge must get cut at the right place and right time, in a process called abscission. The developing cortex is particularly vulnerable to defective abscission: we’ve found that two mutants with loss of abscission genes both have severe microcephaly. These abscission genes are also elevated in several human cancers. Understanding the cellular functions of these genes sheds light on mechanisms of normal and abnormal brain growth, as well as tumor development.
We hypothesize that temporal and spatial regulation of abscission helps expand the early stem cell pool, and influences daughter cell fates. The position of abscission and the midbody remnants may serve important functions in sequestering or transmitting fate determinants. One consequence of defective abscission in the embryonic neural stem cells is p53 elevation and programmed cell death (apoptosis). Interestingly, deletion of p53 prevents the apoptosis and partially rescues microcephaly. Building on these exciting discoveries, we propose to elucidate the in vivo roles of abscission regulation in neural stem cell fates and proper corticogenesis. Using cell biological, genetic, and genomic approaches, we will rigorously dissect the primary effects of abscission dysregulation on cell polarity and fates, as well as the molecular pathways activated.
Axon outgrowth and guidance: how the brain is wired together
Some mutants we study have defects in axon or dendrite formation in post-mitotic neurons. Cortical neurons dissociated from Kif20b mutant brains and cultured in a dish have changes in neuron morphology, associated with abnormalities in the microtubule cytoskeleton. This kinesin motor protein Kif20b plays a role in microtubule cross-linking, as well as localizing molecules within the cell. Our findings add to accumulating evidence suggesting that proteins used to regulate the cytoskeleton during neural stem cell divisions are later used in their neuron daughter cells to regulate neuron shape and growth.