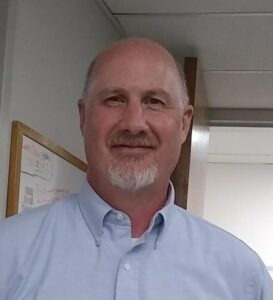
Smith, Jeffrey S.
Primary Appointment
Professor, Biochemistry and Molecular Genetics
Education
- BS, Marine Biology, LIU/ Southampton Campus, Southampton, NY
- PhD, Biochemistry, University of Medicine and Dentistry/ RWJMS
- Postdoc, Molecular Biology, Johns Hopkins School of Medicine
Contact Information
1340 JPA Pinn Hall Room 6229A
PO Box 800733
Charlottesville, VA 22908
Telephone: 434-243-5864
Fax: 434-924-5069
Email: jss5y@virginia.edu
Research Disciplines
Biochemistry, Epigenetics, Genetics, Metabolism, Molecular Biology
Research Interests
Transcriptional Silencing and Aging in Yeast
Research Description
Transcriptional silencing Understanding how chromatin structure influences DNA-mediated processes has become a fundamental problem in cellular biology. Chromatin is known to influence the regulation of eukaryotic gene expression, DNA replication, DNA repair, and recombination. Chromatin effects on gene expression can be either localized (gene-specific) or more regional. The classic example of a regional chromatin effect is silencing, the epigenetic transcriptional repression of large heterochromatic chromosomal loci. Regions of silent chromatin in the budding yeast Saccharomyces cerevisiae include the HML and HMR cryptic mating-type loci, telomeres, and the ribosomal DNA (rDNA). Silencing at each of these loci requires the Silent Information Regulator 2 (SIR2) gene, which encodes the founding member of a large, phylogenetically conserved family of NAD+-dependent protein deacetylases known as the Sirtuins. Sir2 is recruited to the silenced loci in yeast through interactions with other proteins that bind to specific cis-acting silencer elements. Once recruited to chromatin, Sir2 deacetylates lysines on the N-terminal tails of histones H3 and H4, a critical step in the formation of silent chromatin. One of the research projects in our lab is to dissect the mechanism of silencing at the yeast rDNA locus, which was originally defined as the SIR2-dependent repression of Pol II-transcribed reporter genes positioned within the rDNA tandem array. More recently, SIR2 was also found to silence the transcription of endogenous Pol II-transcribed genes and non-coding RNAs embedded in the rDNA. The silent chromatin structure set up by Sir2 also plays a role in suppressing recombination between the rDNA repeats. Interestingly, transcription of the rDNA genes by Pol I (the classic function of the rDNA is to produce rRNA for ribosomes) is required for silencing of Pol II transcription. We are using genetic and biochemical approaches aimed at 1) determining how Pol I transcription promotes silencing of Pol II genes at the rDNA, 2) identifying new genes that function in rDNA silencing, and 3) using rDNA silencing as a model for the study of heterochromatin spreading and boundary element function. In a related project, the lab has been investigating how chromatin modifying enzymes and rDNA chromatin structure, in turn, regulate Pol I-mediated transcription of the rDNA genes. Regulation of rDNA transcription has important implications for diseases such as cancer and cardiac hypertrophy, both of which involve uncontrolled cell growth and require high ribosome translational capacity. NAD+ biosynthesis and the regulation of Sirtuins Sir2 and the other Sirtuins are protein deacetylases that hydrolyze NAD+ as part of their catalytic mechanism. For every deacetylation reaction, one molecule of NAD+ is consumed. The byproducts are nicotinamide and 2'-O-acetyl-ADP ribose, with nicotinamide acting as a strong feedback inhibitor of the reaction. To prevent nicotinamide accumulation and Sirtuin inhibition, yeast cells recycle it into NAD+ though a salvage pathway. This salvage pathway is critical not only for detoxifying the nicotinamide, but to also maintain a cellular NAD+ concentration that is sufficiently high to promote silencing and other Sirtuin-mediated processes. Another area of research in the lab is focused on genetically elucidating new components of the NAD+ biosynthesis and salvage pathways, and determining how they impact on silencing and other Sirtuin regulated processes in yeast such as aging (see below) and thiamine biosynthesis. Information gained from the yeast system is then used as a guide for the investigation of Sirtuin biology in mammalian cells. This is critical because mammalian Sirtuins have been implicated in the regulation of aging-associated diseases including diabetes, cancer, and forms of neurodegeneration. Yeast as a model system for aging and caloric restriction Caloric restriction (CR) is a dietary regiment that extends the lifespan of almost every eukaryotic organism that has been tested, including Saccharomyces cerevisiae. To calorie restrict yeast, we simply reduce the glucose concentration in the growth medium from 2% to 0.5%. This change is sufficient to extend both the replicative lifespan (RLS) and chronological lifespan (CLS) of this organism, where RLS is the number of times that a mother cell divides, and CLS is the number of days that a non-dividing cell remains viable. SIR2 is required for maintaining replicative longevity via its role in controlling rDNA recombination, but is not required for maintaining chronological longevity. We have therefore been utilizing yeast genetics and genomics tools to identify novel genes and cellular pathways that are involved in the control of chronological aging, especially those that are required for the lifespan-extending effects of CR. Such genes and pathways that are conserved in mammals have the potential to be targets for therapeutics in the treatment of age-associated diseases.